Murphy K.R. Biomedical and Veterinary Sciences, Virginia-Maryland College of Veterinary Medicine, Blacksburg, VA, USA
Hay A.N. Department of Small Animal Clinical Sciences, Virginia-Maryland College of Veterinary Medicine, Blacksburg, VA, USA
Rossmeisl Jr. J.H. Department of Small Animal Clinical Sciences, Virginia-Maryland College of Veterinary Medicine, Blacksburg, VA, USA
Klahn S. Department of Small Animal Clinical Sciences, Virginia-Maryland College of Veterinary Medicine, Blacksburg, VA, USA
Vlaisavljevich E. Department of Biomedical Engineering and Mechanics, Virginia Polytechnic Institute and State University, Blacksburg, VA, USA
Tuohy J. Department of Small Animal Clinical Sciences, Virginia-Maryland College of Veterinary Medicine, Blacksburg, VA, USA
Davalos R.V. Department of Biomedical Engineering and Mechanics, Virginia Polytechnic Institute and State University, Blacksburg, VA, USA / Department of Mechanical Engineering, Virginia Polytechnic Institute and State University, Blacksburg, VA, USA / Virginia Tech-Wake Forest University, School of Biomedical Engineering and Sciences, Blacksburg, VA, USA
Coutermarsh-Ott S. Department of Biomedical Sciences and Pathobiology, Virginia-Maryland College of Veterinary Medicine, Blacksburg, VA, USA
Dervisis N.G. Department of Small Animal Clinical Sciences, Virginia-Maryland College of Veterinary Medicine, Blacksburg, VA, USA / Department of Internal Medicine, Virginia Tech Carilion School of Medicine, Roanoke, VA, USA
MeSH keywords:
cat, dog, minimally invasive methods, tumor
Abstract
Cancer in companion animals remains the largest contributor to mortality, with cancer incidence increasing as companion animal longevity continues to improve. Chemotherapy, radiotherapy, and surgery are the main treatment modalities for cancer in veterinary medicine, but these therapies harbor significant limitations. As a result of the limitations of current treatment modalities, significant attention is being paid to the development of minimally-invasive therapeutics for the treatment of companion animal cancers in order to limit morbidity, improve tumor control, and prevent metastasis. In particular, energy-based tumor ablation represents an attractive means of minimally-invasively eradicating tumor tissue, via both thermal and nonthermal mechanisms. Herein, we explore the principles, methodology, and applications of various thermal and nonthermal ablation therapeutics in the treatment of cancer in companion animals. Specifically, we review thermal ablation modalities including cryoablation, high-intensity focused ultrasound (HIFU), laser ablation, microwave ablation, and radiofrequency ablation, as well as nonthermal ablation modalities including histotripsy, irreversible electroporation (IRE), and high-frequency irreversible electroporation (H-FIRE), and evaluate the clinical applications of each in small animal oncology.
Introduction
Cancer represents the largest mortality factor in companion dogs, and partially due to increased longevity of companion animals, the incidence of neoplasia in companion animals continues to rise (Vascellari et al. 2009). The mainstays of cancer therapy in companion animals include surgery, chemotherapy, and radiotherapy. However, surgical resection is frequently precluded due to increased risk for morbidity and potential recovery complications. While chemotherapy and radiotherapy are often employed in an effort to inhibit metastasis, manage clinical symptoms, and overcome surgical care limitations, metastasis of primary tumors remains a major cause of death in veterinary patients. Subsequently, improved cancer therapeutic strategies are urgently needed to improve neoplastic disease management in veterinary medicine.
Special interest is being paid toward the development of minimally-invasive therapeutics in an effort to limit morbidity while maximizing tumor control and preventing metastasis in companion animal cancer patients. Specifically, energy-based tumor ablation is emerging as a rea-listic, efficacious, and potentially cost-effective intervention for cancer in companion animals.
What is Energy-Based Tumor Ablation?
Energy-based tumor ablation therapeutics involve the focal application of energy to destroy tumors, with the goal of eradicating all neoplastic cells within a specified target volume while sparing surrounding healthy tissues. These ablation modalities are administered non- or minimally-invasively, under image-guidance, and can be used as sole treatment modalities, or as adjuvants to surgery, chemotherapy, and radiotherapy. Depending on the ablation modality employed, treatment may induce thermal (Figure 1Α) or nonthermal (Figure 1Β) tumor tissue destruction. Here, we explore the mechanisms, advantages and limitations, and most up-to-date applications of each of various modalities of thermal and nonthermal energy-based ablation of spontaneous tumors in veterinary medicine.
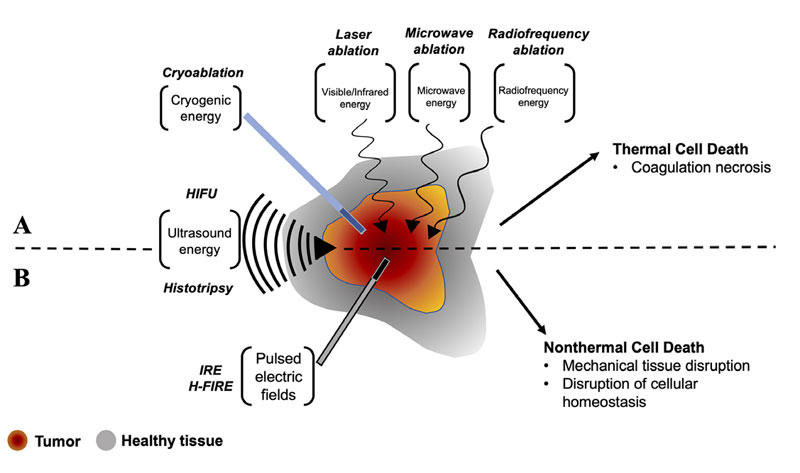
Figure 1. Summary of A) thermal and B) nonthermal energy-based ablation modalities. A) Thermal ablation of tumor tissue can be achieved via hypothermic mechanisms through application of cryogenic energy, or via hyperthermic mechanisms through application of high-intensity focused ultrasound (HIFU) or various frequencies of electromagnetic energy (laser ablation, microwave ablation, radiofrequency ablation). B) Nonthermal ablation of tumor tissue can be achieved through mechanical tissue disruption by application of high-intensity mechanical focused ultrasound (histotripsy), or via disruption of cellular homeostasis after treatment with pulsed electric fields in irreversible electroporation (IRE) and high-frequency irreversible electroporation (H-FIRE).
Thermal Energy-Based Tumor Ablation
Thermal tumor ablation modalities can induce cytotoxic effects via hypo- or hyperthermic mechanisms to induce tissue necrosis. Thermal tumor ablation modalities include cryoablation, laser ablation, microwave ablation (MWA), radiofrequency ablation (RFA), and high-intensity focused ultrasound ablation (HIFU).
Cryoablation
Cryoablation utilizes hypothermal mechanisms to induce cold injury for cytotoxicity. During the procedure, a liquified gas cryogen, most often argon, cools a probe, causing heat to be transferred out of the tissue into the cryoprobe. High pressure helium in the cryoprobe is then used to repeatedly cool and thaw the tissue (Hinshaw et al. 2014). The temperature required to induce cryoablative tissue damage is -20 to -40°C, and these temperatures must extend at least 1 cm beyond the tumor margin in order to fully eliminate the tumor. Cryoablation impacts tissue via direct cell injury via ice ball formation and coagulative necrosis, immunomodulation, vascular injury, and ischemia (Erinjeri & Clark 2010).
Cryoablation allows for easy visualization of the ice ball, correlating with the ablation zone, via ultrasound, CT, or MRI. Additionally, cryoablation is less painful due to the anesthetic effects of cooling nerves and tissues. Each cryoprobe can act independently of other cryoprobes, allowing for very precise tuning of the ablation zone and more customizable treatment. Finally, during cryoablation, although small vessel thrombosis is observed, larger blood vessels are generally spared (Hinshaw et al. 2014). Despite its numerous advantages, cryoablation carries limitations that restrict its applicability in veterinary oncology. First, tumor lysis syndrome and the heat sink effect are possible side effects that may complicate treatment, as well as a possibility of systemic cryoshock (Hinshaw et al. 2014). Additionally, although cryoablation spares large blood vessels, due to the lack of large vessel thrombosis and coagulation, hemorrhagic complications are a concern after cryoablation (Erinjeri & Clark 2010). Treatment can also be limited by cooling efficiency, which is limited to the small surface area around the cryoprobe (Hinshaw et al. 2014). Finally, a particularly notable risk of cryoablation is organ fracture, which can occur in brittle frozen tissues in cases of excessive cryoprobe torque or cryoprobe displacement (Erinjeri & Clark 2010).
The use of cryoablation in veterinary medicine is most reported for skin and subcutaneous tumors, with varying degrees of safety and efficacy noted. In a retrospective study of cryoablation for skin and subcutaneous tumors in dogs, 35% of which were malignant lesions, total ablation was achieved in 94.5% of lesions without relapse or metastasis (Ma et al. 2021).
In one dog, long-term control of post-radiation recurrent nasal adenocarcinoma was reported after CT-guided transnasal cryoablation. Treatment-associated complications included focal lysis of the cribriform plate which self-resolved 13 months post-treatment, and mild chronic nasal discharge. The dog was euthanized 21 months post-treatment due to acute hemoperitoneum, and a sarcoma-consistent hepatic mass was diagnosed at necropsy (Murphy et al. 2011). In one dog with maxillary fibrosarcoma, partial tumor remission was achieved following cryoablation, transarterial embolization, and systemic chemotherapy (Weisse et al. 2011). In a study of ten client-owned dogs with nasal squamous cell carcinoma (SCC), combination CO2 laser ablation and cryosurgery was evaluated for palliation. Following CO2 laser ablation, cryoablation was performed on the visible tumor, as well as adjacent and subadjacent tissue via three rapid freeze/slow thaw cycles. Treatment resulted in an overall survival time of 260 days (Ierace et al. 2018). Tumor recurrence occurred in seven of the ten treated dogs 33-136 days after treatment. Dogs with tumor recurrence were re-treated with combined CO2 laser ablation and cryoablation immediately following recurrence, with three dogs experiencing a second recurrence and one dog experiencing five recurrences. This study demonstrated acceptable palliation of canine nasal SCC, with improved air passage in all dogs and excellent aesthetic outcome (Ierace et al. 2018).
Laser ablation
Laser ablation, often referred to as laser interstitial thermal therapy (LITT), utilizes electromagnetic energy to induce coagulative necrosis via hyperthermic mechanisms. During LITT ablation, lasers deliver specific wavelengths of electromagnetic energy, typically in the near-infrared (IR) range, which is absorbed by tissue-specific chromophores in cells. The cells convert the absorbed energy into heat, which diffuses to adjacent tissues (Hinshaw et al. 2014).
LITT has been used extensively for tumor ablation in veterinary oncology for wide varieties of superficial and deep tumors, including various carcinomas, sarcomas, and adenomas, as well as tumors of the brain, mammary tissue, and prostate, with extremely variable outcomes. In one study of near-IR LITT for thirty-eight dogs with obstruction due to lower urinary track transitional cell carcinoma (TCC), although median survival was 380 days, there were significant risks with the procedure, with complications including stranguria (two dogs), stenosis at the cystourethral junction (three dogs), TCC seeding of the lower urinary tract and urethrostomy site (two dogs), and urethral penetration (one dog) (Cerf & Lindquist 2012).
In two separate studies of the use of transendoscopic LITT for the treatment of Spirocerca lupi-associated esophageal neoplasia in dogs (two and seventeen dogs), LITT ablation was associated with lower morbidity, comparable long-term survival, and shorter hospitalizations compared with surgical management (Yas et al. 2013, Shipov et al. 2015). Another study demonstrated successful endoscopic LITT ablation of a tracheal osteochondroma in a four-month-old golden retriever using a diode laser, with no notable post-treatment complications (Bottero et al. 2016). In these studies, LITT ablation appeared to be a safe and effective palliative treatment option for esophageal and tracheal tumors of various histopathologic subtypes.
LITT ablation has also been applied to superficial tumors in veterinary medicine for both ablation and excision of tumors. In a study of thirty-eight client-owned dogs with a total of forty skin tumors of various types, CO2 lasers were used to compare excision, ablation, and combined excision and ablation laser techniques. Local tumor recurrence occurred in two cases. Healing times was less than twelve days in 88% of dogs treated with the combined excision and ablation protocol, with total procedure times of less than ten minutes (Paczuska et al. 2014). This study demonstrated the advantages of CO2 laser treatment for ablation of superficial tumors, and its potential as an alternative to surgery for skin tumors, as it allows for non-contact excision, simplified procedures, and negligible damage to surrounding tissues (Paczuska et al. 2014).
The combination of LITT tumor ablation and various photosensitizers has been investigated in various veterinary oncologic applications. One study evaluating the cytotoxic effect of intratumoral injection of gold nanorods prior to laser ablation of mammary tumors in ten dogs and six cats showed complete remission in 62.5% of animals, with 25% of animals relapsing after initial treatment response. Notably, gold nanorods had no toxic effect on blood profile or function of the kidney or liver, and overall survival was 315 days (Abdoon et al. 2016). In a separate study, three doses of intratumoral gold nanorods and low-dose LITT at two-week intervals demonstrated that tumors were ablated mainly via apoptosis with a reduction in tumor grade after one treatment and complete regression after three. Metastasis was absent on radiographs one year post-treatment (Ali et al. 2016).
Microwave ablation
Microwave ablation (MWA) is a thermal ablation modality that involves the transmission of microwave electromagnetic energy through an antenna into tumor tissue. The microwave energy results in the orientation of water molecules within an electromagnetic field, which continuously realign when an oscillating microwave field is applied. The continuous realignment of the water molecules increases kinetic energy, raising the temperature of the tissue to cause coagulative necrosis of the tumor (Hinshaw et al. 2014). Similarly to other ablation modalities, MWA is performed under ultrasound or CT image guidance.
The use of MWA may be particularly advantageous for the ablation of high-impedance tumors of the lung and bone, because microwave energy penetrates biological tissues regardless of impedance to electrical current (Andreano & Brace 2013). Further, MWA rapidly achieves high temperatures to reduce treatment times, can create relatively large ablation zones with increased consistency across tissue types, and is relatively insensitive to heat sink effects of large peritumoral blood vessels (Andreano & Brace 2013).
Importantly, there are some limitations of MWA that may reduce its application in veterinary oncology. The necessity of cooling mechanisms in microwave antennas complicates treatment procedures. Additionally, there is a significant clinical learning curve due to the high temperatures and rapid temperature increases achieved during treatment (Andreano & Brace 2013). Finally, MWA is a more recent iteration of thermal tumor ablation and is inherently less explored than other thermal ablation modalities, and therefore clinical data is sparse. Development of improved MWA systems that address these limitations has led to increased application of MWA in humans, which may translate to the veterinary applications. Despite the relatively recent introduction of MWA into veterinary medicine, it has been limitedly explored for applications in the ablation of tumors of higher impedance tissues such as the liver, lung, kidney, and bone.
In a study of five dogs with nonresectable primary and metastatic liver tumors, MWA was performed without procedural complications (Yang et al. 2017). However, assessing survival benefit and disease-free periods were not within the scope of this study. In a study including two dogs with metastatic hemangiosarcoma of the liver and primary hepatocellular carcinoma, technical feasibility of laparoscopic MWA was established, with no procedural complications (Oramas et al. 2019). This study also probed six canine cadavers to confirm that all liver lobes were laparoscopically accessible for MWA, suggesting that laparoscopic MWA may be a feasible, minimally-invasive treatment option for nonresectable hepatic neoplasia. Percutaneous MWA has also been successfully applied for the treatment of renal carcinoma in one dog, with no complications occurring and the dog still alive 3 years post-treatment (Culp et al. 2017).
MWA has also been explored for the treatment of both metastatic and primary appendicular osteosarcoma. In a 10-year old mixed breed dog with pulmonary metastasis secondary to appendicular osteosarcoma, video-assisted MWA was performed thoracoscopically without complication (Mazzaccari et al. 2017). The dog lived for 257 days after the procedure with improved clinical signs after treatment, however the patient had previously undergone doxorubicin and carboplatin chemotherapy prior to the diagnosis of the pulmonary lesion, and it is likely that the observed survival benefit after MWA is partially attributable to the chemotherapy. In another study of two dogs with appendicular osteosarcoma with presumptive pulmonary metastasis undergoing chemotherapy, Dornbush et al. performed percutaneous MWA under ultrasound and CT image guidance (Dornbusch et al. 2020). In this study, both dogs sustained mild and moderate pneumothorax during the procedure, with survival times that were comparable to dogs that did not receive therapy. However, the procedure was 50-66% as expensive as a thoracotomy with lung lobectomy, suggesting that MWA of lung metastasis may be significantly more cost effective than surgery.
The use of MWA for the treatment of bone tumors is of particular interest, due to the high impedance of bone that limits application of other thermal ablation modalities. MWA for the treatment of neoplastic bone has been limitedly explored, with extremely variable clinical responses. In the first veterinary pilot study of MWA for bone tumors, six dogs with distal radial osteosarcoma were treated with fluoroscopy-guided MWA (Salyer et al. 2020). There were no procedural complications, but long-term follow up on clinical signs was not reported. Tumor necrosis post-treatment varied significantly between 30 and 90%, necessitating larger sample sizes and longer follow-ups to further assess applicability of MWA for the treatment of bone tumors in dogs.
Radiofrequency ablation
Radiofrequency ablation (RFA) applies alternating electrical currents (200-1, 200 Hz) between interstitial electrodes, to activate the Joule effect. Under the Joule effect, flow of the electric current causes ions in adjacent tissues to rapidly oscillate, which raises tissue temperature near the electrodes via friction (Hong & Georgiades 2010). The resulting thermal ablation zone can then grow via thermal diffusion and conduction. RFA can be monopolar, in which current is transmitted through an interstitial electrode and returns through grounding pads on the skin, or bipolar, in which current oscillates between two interstitial electrodes in the absence of a grounding pad (Hong & Georgiades 2010).
Despite the minimally-invasive nature of RFA and customizability of treatment with electrode configurations, there are significant setbacks to this modality that limit its application in veterinary medicine. Dermal wounds can easily occur during treatment if there are insufficient quantities or uneven placement of grounding pads (Rhim et al. 2004). The nature of RFA also limits its application to certain tissue types. Specifically, heat transfer via conduction is slow and struggles to overcome local tissue perfusion and ventilation in most tissues, which leads to significant variation in RFA zones depending on the tissue heterogeneity.
In addition to applications for the treatment of hyperthyroidism (Mallery et al. 2003) and hyperparathyroidism (Pollard et al. 2001), the majority of the use of RFA in veterinary oncology has been in experimental tumor models, with limited case reports in spontaneous disease. To the best of the authors’ knowledge, the only cases of the use of RFA for the ablation of spontaneous tumors in veterinary patients are for prostatic carcinoma and cardiac chemodectomas. In the case of one dog diagnosed with prostatic carcinoma, RFA was performed intraoperatively via direct visualization of the prostate tumor, with successful ablation and no complications (Culp et al. 2017). In the first description of percutaneous echo-guided RFA of aortic tumors, ultrasound-guided RFA was used to treat chemodectomas in five dogs, yielding no complications during the procedure or in the following 24 hours (Gomez Ochoa et al. 2021). Procedures were generally 35 minutes long with discharge times of two hours, suggesting that echo-guided RFA may be a robust and cost-effective method for treatment of cardiac tumors in dogs. There was a marked reduction in tumor size in all dogs one month after treatment, and these size reductions were only slight three months after treatment, suggesting incomplete ablation. However, one dog was treated with a second percutaneous echo-guided RFA eight months later to relieve vena cava compression, and the dog remained stable 6 months after this procedure, suggesting that serial echo-guided RFA may be feasible for the treatment of canine chemodectomas (Gomez Ochoa et al. 2021).
High-intensity focused ultrasound (HIFU) ablation
HIFU ablation involves the delivery of high power, converging ultrasound beams from an extracorpeal ultrasound transducer into targeted tissue volumes (Figure 2). The mechanical vibration of the focused sound waves generates heat in the tissue, causing coagulative necrosis of target tissue within the focal plane of the ultrasound beam (Ter Haar 2016). HIFU ablation is performed under MR or ultrasound image guidance, and is considered the least invasive thermal ablation modality when using an extracorpeal transducer for the ablation of superficial and benign tumors not surrounded by bone and air (Knavel & Brace 2013). HIFU can also be performed with transrectal, interstitial, and percutaneous transducers for the ablation of prostate tumors, biliary and esophageal tumors, and deeper tumors with limitations, respectively (Ter Haar 2016).
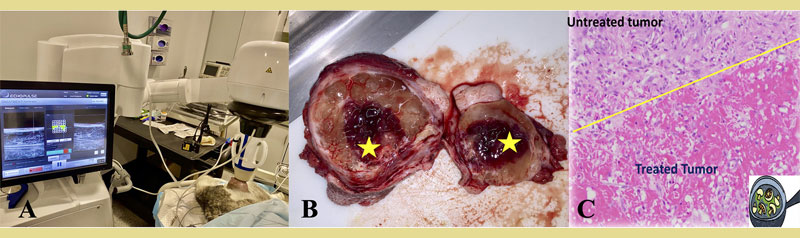
Figure 2. High-intensity focused ultrasound (HIFU) treatment of subcutaneous tumors in canine patients. A) A dog diagnosed with a low grade soft tissue sarcoma is under inhalational general anesthesia. A clinical ultrasound-guided HIFU device is used to target the tumor located at the left lateral thigh of the dog. The treatment unit is positioned over the targeted tumor, and the treatment is monitored in real time via ultrasonography. (B) Gross image of a HIFU-treated tumor. The yellow star indicates the treated portion of the tumor, characterized by diffuse hemorrhage and dark red to black color. (C) Microscopy image of the treated tumor stained with standard H&E, demonstrating coagulative necrosis and haemorrhage at the treated site, sharply delineated from the untreated tumor tissue (yellow line). (source of the images: personal authors’ file)
In addition to being the only completely non-invasive thermal ablation modality, HIFU ablation can generally be performed with high precision between treated and untreated tissue. However, HIFU ablation is potentially less effective for deeper tumors because of low penetrance of ultrasound through tissues (Ter Haar 2016). Additionally, there is risk of skin burns, peripheral nerve damage, and intestinal injury due to scatter and reflection of ultrasound waves, particularly when treating in areas obstructed by bone, that lead to off-target beam focus (Seward et al. 2019). HIFU ablation is further limited in its use in areas that are subject to respiratory motion due to decreased precision, or are shielded by bone (Seward et al. 2019).
HIFU ablation has a wide range of clinical application for tumor ablation in veterinary oncology, but many studies are retrospective in nature and there have been few controlled clinical trials of the use of HIFU tumor ablation in veterinary patients.
Ryu et al. report a retrospective study of eleven dogs that underwent HIFU ablation of solid tumors, which included multiple transitional cell carcinomas of the bladder, as well as carcinomas and mast cell tumors of the mammary gland, orbit, hip, and abdominal cavity (Ryu et al. 2018). The majority of truncal and axillary tumors and all extremity tumors were targetable with HIFU, while head and spinal tumors were the least targetable tumor types. Clinical signs improved in five of the treated dogs, while stopping bleeding in four dogs with hemorrhagic tumors despite some complications including hyperthermia, erythema, enteritis, and skin burns. The optimal treatment volume for HIFU ablation of STS was determined to be ≤200 cm3, with optimal and maximum target depths of ≤8 cm and ≤11 cm, respectively. In another retrospective study of dogs with soft tissue sarcoma, based on imaging analysis, accessibility of the tumor site by ultrasound beam, and proximity to the spinal cord, Seward et al. report that 81% of dogs diagnosed with soft tissue sarcoma had tumors targetable with MR-guided HIFU ablation. Head and spinal tumors were least likely to be targetable, but all extremity tumors and most truncal and axillary tumors were considered targetable, suggesting potentially wide clinical application of HIFU ablation for the treatment of canine soft tissue sarcoma.
Clinical trial evaluation of HIFU ablation of spontaneous tumors in veterinary patients is limited, but a number of small studies have been reported evaluating HIFU ablation for a variety of tumor types, both superficial and deep. Kopelman et al. investigated the use of MR-guided HIFU for the ablation of hepatocellular carcinoma in one dog, applying four separate treatments over eight weeks. Following excision of the tumor after the final HIFU treatment, HIFU appeared to target the tumor with high precision but induced focal thermal injury to the cartilage of the right lower ribs, causing infection requiring surgical intervention and negating the noninvasive nature of the HIFU ablation (Kopelman et al. 2006). Although the patient was healthy ten months post-treatment, this study highlights the potential risks of applying HIFU ablation to deep-seated tumors. HIFU ablation has been shown to successfully induce total tumor remission of a large oral neurilemmoma of the left maxilla of one canine patient, despite some procedural adverse events including buccal mucosa burns requiring surgical and hyperbaric oxygen management (Ranjan et al. 2021). While suggesting that HIFU ablation may circumvent the need for surgical resection of bony tissue in the treatment of canine oral cancer, this study also identified elevated proliferation of T cells in tumor and blood samples, suggesting that HIFU ablation may further its antitumor effect via engagement of the immune system. In a clinical trial of HIFU ablation of spontaneous tumors in veterinary patients, superficial tumors of the neck, leg, face, back, and belly were treated with MR-guided HIFU in six dogs and four cats (Antoniou et al. 2022). Coagulative necrosis of the tumor was evident in 80% of cases, but lower magnification histological images falsely suggested total destruction of all cancer cells, while higher magnification images revealed intact cancer cells. This suggests that HIFU may be most effective when used as an adjunct to radiation therapy or chemotherapy in order to ensure total elimination of tumor cells. In the largest clinical trial of HIFU ablation of spontaneous tumors in veterinary patients to date, Carroll et al. performed HIFU for partial tumor ablation in twenty dogs diagnosed with subcutaneous solid tumors, including fifteen soft tissue sarcomas, three mast cell tumors, one osteosarcoma, and one thyroid carcinoma. HIFU treatment was well-tolerated, with only one dog experiencing a clinically significant adverse event. A degranulation event occurred in a patient diagnosed with a mast cell tumor, which was restricted to the tumor site and subsequently addressed during the planned surgical resection post-HIFU. Tumors were resected three to six days after HIFU treatment, with complete tissue ablation and immune cell infiltration evident on pathology and immunohistochemistry. Additionally, this study identified an increase in the expression of T-cell activation-related genes post-HIFU ablation, suggesting that HIFU may induce immunostimulatory changes to modulate the tumor microenvironment (Carroll et al. 2022). Ablation of the entire tumor was not a goal of this study, and therefore additional work is needed to assess survival and clinical benefit of HIFU ablation of subcutaneous tumors, both as a sole treatment modality and as an adjunct to standard therapies for the treatment of subcutaneous tumors in dogs and cats.
Nonthermal Energy-Based Ablation in Veterinary Medicine
Energy-based tumor ablation can also be achieved via nonthermal mechanisms. In nonthermal tumor ablation modalities, energy is still applied to tumors, but rather than inducing temperature changes in the tissue to kill cells via thermal mechanisms, these modalities apply energy to manipulate cellular properties or mechanically disrupt target cells to kill cells via nonthermal mechanisms. By not relying on temperature changes within tissue to kill neoplastic cells, which are dependent on tissue properties that are highly heterogenous in neoplastic tissue, nonthermal tumor ablation may achieve more uniform ablation and be more widely applicable across various tissue types and near critical structures. Nonthermal ablation modalities of interest in veterinary medicine include histotripsy, irreversible electroporation (IRE), and high-frequency irreversible electroporation (H-FIRE).
Histotripsy
Histotripsy is a recently developed noninvasive, non-ionizing, mechanical, cavitation-based therapy that employs pulsed high-intensity focused ultrasound waves for the nonthermal destruction of targeted tissues (Bader et al. 2019, Xu et al. 2021). Histotripsy involves the delivery of very high amplitude, short-duration ultrasound pulses, which generate a dense, energetic bubble cloud in the tissue, resulting in mechanical tissue fractionation following repeated expansion and collapse of bubbles of endogenous gases in tissue upon interaction with the ultrasound beam (Vlaisavljevich et al. 2016b). These cavitation microbubbles are thought to form in the extracellular matrix, the rapid expansion and collapse of which produces high strain and stress on adjacent cells (Vlaisavljevich et al. 2016b), effectively liquifying the target tissue into a homogenate of acellular debris to be reabsorbed by the body over 1-2 months (Vlaisavljevich et al. 2016a).
Tissue-damage thresholds for histotripsy are tissue-specific (Vlaisavljevich et al. 2014), indicating potential for treatment optimization, tumor-specific ablation, and sparing of healthy tissue. Because cell death by histotripsy occurs by binary mechanisms, with cellular damage only occurring when ultrasound pressure exceeds the cavitation threshold, histotripsy ablation results in very sharp boundaries between ablated and unablated tissue, with transition zone of partial ablation only spanning a few hundred microns (Maxwell et al. 2013). This nonthermal mechanism of histotripsy ablation overcomes many limitations associated with thermal ablation, such as the heat sink effect, lack of precise margins, and predictability of therapy (Xu et al. 2019). The feasibility of using histotripsy as a blood vessel-sparing ablation technique has also been shown in porcine liver in vivo (Vlaisavljevich et al. 2014). Because histotripsy uses extracorporeal transducers, tumor ablation can be performed noninvasively. Additionally, the liquification of the tumor eliminates need for tumor excision, thus reducing risk of surgery-associated complications such as thrombosis (Xu et al. 2021).
The applicability of histotripsy for ablation of tumors in gas-rich organs, such as the lung and gastrointestinal tract, is limited due to the exceptionally low cavitation thresholds in these tissues which could result in significant off-target effects (Xu et al. 2021). Additionally, the application of histotripsy in areas significantly blocked by bone is limited (Xu et al. 2021).
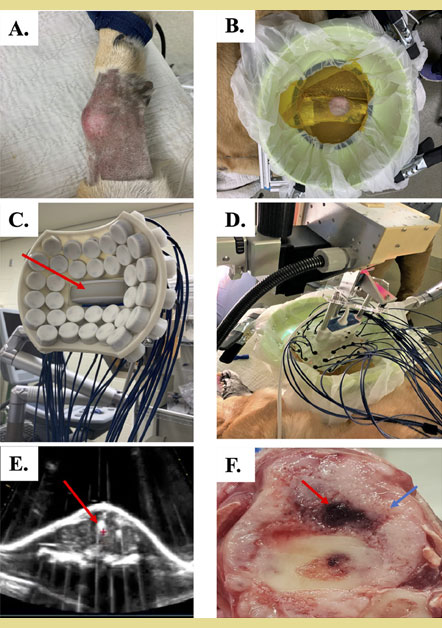
Figure 3. Histotripsy treatment setup and treatment of canine osteosarcoma
A) Primary appendicular osteosarcoma on the distal radius of a canine patient. Hair covering the skin over the tumor was removed in preparation for histotripsy treatment.
B) Histotripsy treatment coupling bowl filled will degassed water placed over the tumor.
C) 500kHz 32-element histotripsy transducer with ultrasound probe (red arrow) co-axially aligned for real-time imaging during treatment delivery.
D) Transducer and treatment bowl positioned together and ready to deliver treatment.
E) Cavitation bubble cloud generated during histotripsy treatment (indicated by red arrow).
F) Gross pathology of treated primary bone tumor, with red arrow indicating treatment site, and blue arrow indicating untreated tumor
Due to the novelty of the histotripsy technology for tumor ablation, its applications in veterinary medicine are limited but accelerating. Proof of principle studies demonstrate efficacy of histotripsy in ablating excised canine osteosarcoma tumors (Arnold et al. 2021). Additionally, in the first clinical trial of histotripsy for partial ablation of osteosarcoma in five dogs, successful ablation was achieved within target treatment volumes as evidenced by gross pathology and histology, with no significant clinical adverse events (Ruger et al. 2022b). A first in patient pilot study of the same technology has been completed for the treatment of canine soft tissue sarcomas, demonstrating the feasibility, safety, and treatment-induced tumor microenvironment changes (Ruger et al. 2022a). These studies established safety and feasibility of histotripsy for non-invasive treatment of primary bone tumors (Figure 3) and soft tissue sarcomas (Figure 4) in dogs, but additional work is needed to evaluate long-term efficacy and survival benefit. Additional ongoing clinical trials continue to investigate histotripsy for the ablation of canine brain tumors and feline soft tissue sarcoma.
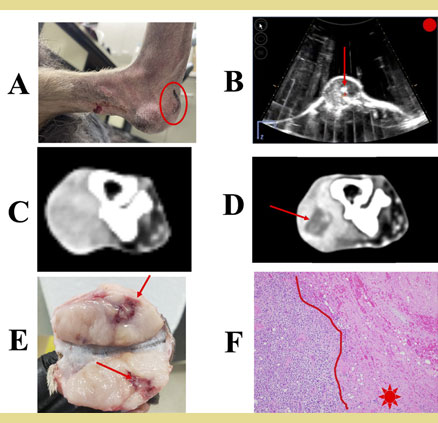
Figure 4. Mechanical, high-intensity focused ultrasound ablation of a ca- nine soft tissue sarcoma with histotripsy.
A) Soft tissue sarcoma on the plantar aspect of the left metatarsal, denoting the treatment site (circled).
B) Live image of histotripsy treatment illustrating the cavitation bubble cloud within the tumor (arrow).
C) Contrast-enhanced CT images before treatment and
D) 1 day post-treatment with histotripsy denoting the lack of contrast enhancement in the ablation zone (arrow).
E) Gross image denoting hemorrhage (arrow), and
F) histopathology image denoting complete loss of cell viability within a sharply-demarcated ablation zone (star).
Irreversible Electroporation (IRE) and High-Frequency Irreversible Electroporation (H-FIRE)
During irreversible electroporation (IRE), cells are exposed to monopolar pulsed electric fields that elevate the transmembrane potential of target cells, destabilize the cell membrane, and induce nonthermal cell death (Davalos et al. 2005). Electric fields are generated and pulsed through target tissues between two needle electrodes inserted into tumors. The second generation of IRE, high-frequency irreversible electroporation (H-FIRE), delivers high-frequency, bipolar pulsed electric fields to induce sharply demarcated regions of nonthermal ablation. Compared to IRE, H-FIRE allows for simpler procedures as it does not require the need for neuromuscular blockade or cardiac synchronization, and reduces the number of electrodes needed for treatment (Arena et al. 2011).
The nonthermal mechanisms of IRE and H-FIRE permit ablation near heat-sensitive structures, and for preservation of nerves and blood vessels enabled by careful placement of needle electrodes. IRE and H-FIRE tumor ablation is also minimally invasive, and has been shown to elicit anti-tumor adaptive immune response (Ringel-Scaia et al. 2019). Additionally, H-FIRE preferentially ablates neoplastic cells while sparing healthy cells at the tumor margin, allowing for precise tumor ablation (Ivey et al. 2015). However, the insertion and removal of needle electrodes into a tumor poses risk of neoplastic cell seeding along the electrode tracks during IRE and H-FIRE tumor ablation.
Figure 5. Stereotactic, image-guided ablation of a canine high-grade glioma with irreversible electroporation (IRE). Parasagittal, post-contrast T1W MRI (A) and intraoperative post-contrast CT (B) illustrating the enhancing tumor in the frontal lobe with probe guide pedestal (PGP) implanted in the overlying frontal bone (arrow, A). Fusion of MRI and intraoperative CT images (C) demonstrating in situ placement of two electrodes (black arrows) used for ablation through the PGP into the tumor.
IRE (Figure 5) and H-FIRE (Figure 6) have been employed in a number of small case studies for tumor ablation in spontaneous canine neoplasia. IRE has been used for the ablation of soft tissue sarcoma in one canine patient (Neal et al. 2011), and for the ablation of canine glioma both as a sole treatment modality (Rossmeisl et al. 2015) and with adjuvant radiotherapy (Garcia et al. 2011). When IRE was employed for the ablation of a periarticular histiocytic sarcoma of the hindlimb, clinical signs of lameness improved, but tumor relapse occurred. The dog received a CCNU chemotherapy regimen and was re-treated with IRE to address focal relapse four months post-treatment presumably due to CCNU resistance, and total tumor remission was achieved (Neal et al. 2011). The dog survived for an additional 5 years post treatment and was euthanized due to unrelated causes. This study established the potential application of IRE for the treatment of primary neoplasms, as well as for the treatment of tumors refractory to other therapeutics. Garcia et al. later employed IRE with adjuvant fractionated radiotherapy for multimodal treatment of spontaneous canine glioma in one patient, resulting in a 75% reduction in tumor size 48 hours post-IRE and significant improvement in clinical signs in the weeks following IRE throughout the course of radiotherapy (Garcia et al. 2011). Complete remission was documented four months post-IRE, despite evidence of early-delayed radionecrosis eventually necessitating euthanasia 149 days post-IRE, with no evidence of tumor recurrence at necropsy. Taken together, it is possible that IRE treatment radiosensitized glioma in this patient, which warrants further investigation. In a later study of seven dogs with spontaneous glioma treated with IRE alone, severe post-treatment toxicity, including fatal aspiration pneumonia and treatment-associated cerebral edema, occurred in two dogs. However, clinical signs improved uniformly, with survival times up to 940 days post-IRE with a median survival time of 119 days (Rossmeisl et al. 2015).
Figure 6. Use of high-frequency irreversible electroporation (H-FIRE) for ablation of canine soft tissue tumors. A) Combinations of pulsing sequences across two bipolar H-FIRE electrodes can achieve a spatially larger, convergent tissue ablation zone. B) Parallel, bipolar H-FIRE probes held with predetermined distance between them via 3D-printed guide (left) are inserted into a primary liver tumor, using an open approach surgical procedure (middle). Insertion of a single bipolar electrode for targeted ablation of multiple nodules at the surface of the liver (right).
Procedural limitations and treatment-related complications of IRE tumor ablation in veterinary medicine prompted the development of H-FIRE, which has been investigated for the ablation of spontaneous canine meningioma and hepatocellular carcinoma. In a study of H-FIRE ablation of spontaneous meningioma in three dogs, Latouche et al. performed H-FIRE without intra- or postoperative complication in two of three dogs, with resection-associated hemorrhage and hypotension occurring in one dog (Latouche et al. 2018). Uniform necrotic ablations were achieved in two of three dogs, but nonuniform treatment occurred in one dog, likely due to intratumoral mineralization. One dog remained tumor-free six months post-treatment, and tumor recurrence was suspected in another dog five months post-H-FIRE (Latouche et al. 2018). This study established safety and clinical feasibility of the intracranial H-FIRE procedure, but due to the treat-and-resect nature of this study, additional work is needed in order to evaluate long-term efficacy of H-FIRE in inducing tumor remission for dogs diagnosed with meningioma. In a later study of H-FIRE for the ablation of hepatocellular carcinoma in three dogs, Partridge et al. demonstrated well-defined percutaneous ablation in two out of three patients, with mild muscle contraction and no cardiac arrhythmia, and without clinically significant treatment-related adverse events (Partridge et al. 2020). Infiltration of CD3+ lymphocytes was observed in reactive zones surrounding treated tumor volumes, suggesting the induction of a pro-inflammatory immune response after H-FIRE ablation of hepatocellular carcinoma. Two of three dogs were still alive more than twelve months after treat-and-resect H-FIRE treatment, with no evidence of tumor recurrence or metastasis (Partridge et al. 2020). Additional work is needed in order to evaluate the survival benefit and long-term antitumor effects of H-FIRE in the ablation of spontaneous canine hepatocellular carcinoma without tumor resection, but this study established safety and feasibility of the procedure with promising clinical results. Investigations of the use of H-FIRE for the treatment of primary lung tumors in dogs are ongoing.
Conclusions
Energy-based tumor ablation warrants further investigation in veterinary medicine, as it demonstrates particular promise in offering both curative and palliative treatment to companion animals with cancer. Energy-based ablation has the potential to offer outpatient, minimally- or non-invasive, precise, and customizable antineoplastic therapy, while limiting morbidity associated with standard treatment modalities. The widespread use of energy-based tumor ablation therapeutics in veterinary medicine is in its early stages, largely due to technical expertise needed and cost of new instrumentation systems. With further investigation, both thermal and nonthermal energy-based tumor ablation modalities can be optimized and applied as sole therapeutics or as adjuvants to standard treatment modalities for the treatment of diverse tumor types in veterinary oncology.
Conflicts of Interest
Drs Dervisis, Rossmeisl, and Davalos have issued patents and/or patents pending in the area of irreversible electroporation. Drs Dervisis and Davalos are serving as scientific advisors with ManaMedTech LLC. Dr. Vlaisavljevich has a financial interest and ongoing research partnership with HistoSonics Inc.
Acknowledgements
We would like to extend a heartfelt thanks to all of the dedicated clients/pet parents and their primary care veterinarians for actively seeking to enroll their animals in the various clinical trials summarized in this manuscript. Without them, there can be no advances in the field of veterinary cancer medicine.
References
- Abdoon AS, Al-Ashkar EA, Kandil OM, Shaban AM, Khaled HM, El Sayed MA, El Shaer MM, Shaalan AH, Eisa WH, Eldin AA, Hussein HA, El Ashkar MR, Ali MR, Shabaka AA (2016) Efficacy and toxicity of plasmonic photothermal therapy (PPTT) using gold nanorods (GNRs) against mammary tumors in dogs and cats. Nanomedicine 12, 2291-2297.
- Ali MR, Ibrahim IM, Ali HR, Selim SA, El-Sayed MA (2016) Treatment of natural mammary gland tumors in canines and felines using gold nanorods-assisted plasmonic photothermal therapy to induce tumor apoptosis. Int J Nanomedicine 11, 4849-4863.
- Andreano A, Brace CL (2013) A comparison of direct heating during radiofrequency and microwave ablation in ex vivo liver.Cardiovasc Intervent Radiol 36, 505-11.
- Antoniou A, Evripidou N, Panayiotou S, Spanoudes K, Damianou C (2022) Treatment of canine and feline sarcoma using MR-guided focused ultrasound system. J Ultrasound.
- Arena CB, Sano MB, Rossmeisl JH Jr., Caldwell JL, Garcia PA, Rylander MN, Davalos RV (2011) High-frequency irreversible electroporation (H-FIRE) for non-thermal ablation without muscle contraction. Biomed Eng Online 10, 102.
- Arnold L, Hendricks-Wenger A, Coutermarsh-Ott S, Gannon J, Hay AN, Dervisis N, Klahn S, Allen IC, Tuohy J, Vlaisavljevich E (2021) Histotripsy Ablation of Bone Tumors: Feasibility Study in Excised Canine Osteosarcoma Tumors. Ultrasound Med Biol 47, 3435-3446.
- Bader KB, Vlaisavljevich E, Maxwell AD (2019) For Whom the Bubble Grows: Physical Principles of Bubble Nucleation and Dynamics in Histotripsy Ultrasound Therapy. Ultrasound Med Biol 45, 1056-1080.
- Bottero E, Cagnasso A, Gianella P (2016) Diode laser ablation of a tracheal osteochondroma in a dog. J Small Anim Pract 57, 382-5.
- Carroll J, Coutermarsh-Ott S, Klahn SL, Tuohy J, Barry SL, Allen IC, Hay AN, Ruth J, Dervisis N (2022) High intensity focused ultrasound for the treatment of solid tumors: a pilot study in canine cancer patients. Int J Hyperthermia 39, 855-864.
- Cerf DJ, Lindquist EC (2012) Palliative ultrasound-guided endoscopic diode laser ablation of transitional cell carcinomas of the lower urinary tract in dogs. J Am Vet Med Assoc 240, 51-60.
- Culp W, Johnson E, Palm C. Use of thermal ablation techniques in the treatment of canine urogenital neoplasia. In: Proceedings of Veterinary Interventional Radiology and Interventional Endoscopy Society Meeting 2017, Cabo San Lucas, Mexico, p. 6.
- Davalos RV, Mir IL, Rubinsky B (2005) Tissue ablation with irreversible electroporation.Ann Biomed Eng 33, 223-31.
- Dornbusch JA, Wavreille VA, Dent B, Fuerst JA, Green EM, Selmic LE (2020) Percutaneous microwave ablation of solitary presumptive pulmonary metastases in two dogs with appendicular osteosarcoma. Vet Surg 49, 1174-1182.
- Erinjeri JP, Clark TW (2010) Cryoablation: mechanism of action and devices. J Vasc Interv Radiol 21, 187-91.
- Garcia PA, Pancotto T, Rossmeisl JH, Jr., Henao-Guerrero N, Gustafson NR, Daniel GB, Robertson JL, Ellis TL, Davalos RV (2011) Non-thermal irreversible electroporation (N-TIRE) and adjuvant fractionated radiotherapeutic multimodal therapy for intracranial malignant glioma in a canine patient. Technol Cancer Res Treat 10, 73-83.
- Gomez Ochoa P, Alferez MD, de Blas I, Fernendes T, Sanchez Salguero X, Balana B, Melendez Lazo A, Barbero Fernandez A, Caivano D, Corda F, Corda A (2021) Ultrasound-Guided Radiofrequency Ablation of Chemodectomas in Five Dogs. Animals (Basel) 11.
- Hinshaw JL, Lubner MG, Ziemlewicz TJ, Lee FT, Jr., Brace CL (2014) Percutaneous tumor ablation tools: microwave, radiofrequency, or cryoablation-what should you use and why? Radiographics 34, 1344-62.
- Hong K, Georgiades C (2010) Radiofrequency ablation: mechanism of action and devices. J Vasc Interv Radiol 21, S179-86.
- Ierace MK, Canfield MS, Peters-Kennedy J, Kane CW (2018) Combined carbon dioxide laser and cryosurgical ablation of rostral nasal septum squamous cell carcinoma in 10 dogs. Vet Dermatol 29, 431-e142.
- Ivey JW, Latouche EL, Sano MB, Rossmeisl JH, Davalos RV, Verbridge SS (2015) Targeted cellular ablation based on the morphology of malignant cells. Sci Rep 5, 17157.
- Knavel EM, Brace CL (2013) Tumor ablation: common modalities and general practices. Tech Vasc Interv Radiol 16, 192-200.
- Kopelman D, Inbar Y, Hanannel A, Dank G, Freundlich D, Perel A, Castel D, Greenfeld A, Salomon T, Sareli M, Valeanu A, Papa M (2006) Magnetic resonance-guided focused ultrasound surgery (MRgFUS). Four ablation treatments of a single canine hepatocellular adenoma. HPB (Oxford) 8, 292-8.
- Latouche EL, Arena CB, Ivey JW, Garcia PA, Pancotto TE, Pavlisko N, Verbridge SS, Davalos RV, Rossmeisl JH (2018). High-Frequency Irreversible Electroporation for Intracranial Meningioma: A Feasibility Study in a Spontaneous Canine Tumor Model. Technol Cancer Res Treat 17, 1533033818785285.
- Ma J, Yu X, Lv J, Lin D, Lin J, Bai Y, Wang Y, Li X, Dong J (2021) Cryotherapy mediates histopathological and microstructural changes during the treatment of skin and subcutaneous tumors in dogs. Cryobiology 98, 164-171.
- Mallery KF, Pollard RE, Nelson RW, Hornof WJ, Feldman EC (2003) Percutaneous ultrasound-guided radiofrequency heat ablation for treatment of hyperthyroidism in cats. J Am Vet Med Assoc 223, 1602-7.
- Maxwell AD, Cain CA, Hall TL, Fowlkes JB, Xu Z (2013) Probability of cavitation for single ultrasound pulses applied to tissues and tissue-mimicking materials. Ultrasound Med Biol 39, 449-65.
- Mazzaccari K, Boston SE, Toskich BB, Bowles K, Case JB (2017) Video-assisted microwave ablation for the treatment of a metastatic lung lesion in a dog with appendicular osteosarcoma and hypertrophic osteopathy. Vet Surg 46, 1161-1165.
- Murphy SM, Lawrence JA, Schmiedt CW, Davis KW, Lee FT, Jr., Forrest LJ, Bjorling DE (2011) Image-guided transnasal cryoablation of a recurrent nasal adenocarcinoma in a dog. J Small Anim Pract 52, 329-33.
- Neal RE, 2nd, Rossmeisl JH, Jr., Garcia PA, Lanz OI, Henao-Guerrero N, Davalos RV (2011) Successful treatment of a large soft tissue sarcoma with irreversible electroporation. J Clin Oncol 29, e372-7.
- Oramas A, Case JB, Toskich BB, Fox-Alvarez WA, Boston SE, Giglio RF, Dark MJ (2019) Laparoscopic access to the liver and application of laparoscopic microwave ablation in 2 dogs with liver neoplasia. Vet Surg 48, 91-98.
- Paczuska J, Kielbowicz Z, Nowak M, Antonczyk A, Ciaputa R, Nicpon J (2014) The carbon dioxide laser: an alternative surgery technique for the treatment of common cutaneous tumors in dogs. Acta Vet Scand 56, 1.
- Partridge BR, O’Brien TJ, Lorenzo MF, Coutermarsh-Ott SL, Barry SL, Stadler K, Muro N, Meyerhoeffer M, Allen IC, Davalos RV, Dervisis NG (2020) High-Frequency Irreversible Electroporation for Treatment of Primary Liver Cancer: A Proof-of-Principle Study in Canine Hepatocellular Carcinoma. J Vasc Interv Radiol 31, 482-491 e4.
- Pollard RE, Long CD, Nelson RW, Hornof WJ, Feldman EC (2001) Percutaneous ultrasonographically guided radiofrequency heat ablation for treatment of primary hyperparathyroidism in dogs. J Am Vet Med Assoc 218, 1106-10.
- Ranjan A, Kishore D, Ashar H, Neel T, Singh A, More S (2021) Focused ultrasound ablation of a large canine oral tumor achieves efficient tumor remission: a case report. Int J Hyperthermia 38, 552-560.
- Rhim H, Dodd GD, 3rd, Chintapalli KN, Wood BJ, Dupuy DE, Hvizda JL, Sewell PE, Goldberg SN (2004) Radiofrequency thermal ablation of abdominal tumors: lessons learned from complications. Radiographics 24, 41-52.
- Ringel-Scaia VM, Beitel-White N, Lorenzo MF, Brock RM, Huie KE, Coutermarsh-Ott S, Eden K, McDaniel DK, Verbridge SS, Rossmeisl JH, Jr., Oestreich KJ, Davalos RV, Allen IC (2019) High-frequency irreversible electroporation is an effective tumor ablation strategy that induces immunologic cell death and promotes systemic anti-tumor immunity. EBioMedicine 44, 112-125.
- Rossmeisl JH, Jr., Garcia PA, Pancotto TE, Robertson JL, Henao-Guerrero N, Neal RE, 2nd, Ellis TL, Davalos RV (2015) Safety and feasibility of the NanoKnife system for irreversible electroporation ablative treatment of canine spontaneous intracranial gliomas. J Neurosurg 123, 1008-25.
- Ruger L, Yang E, Gannon J, Sheppard H, Coutermarsh-Ott S, Ziemlewicz TJ, Dervisis N, Allen IC, Daniel GB, Tuohy J, Vlaisavljevich E, Klahn S (2022a) Mechanical High-Intensity Focused Ultrasound (Histotripsy) in Dogs with Spontaneously Occurring Soft Tissue Sarcomas. IEEE Trans Biomed Eng PP.
- Ruger LN, Hay AN, Gannon JM, Sheppard HO, Coutermarsh-Ott SL, Daniel GB, Kierski KR, Ciepluch BJ, Vlaisavljevich E, Tuohy JL (2022b) Histotripsy Ablation of Spontaneously Occurring Canine Bone Tumors In Vivo. IEEE Trans Biomed Eng PP.
- Ryu MO, Lee SH, Ahn JO, Song WJ, Li Q, Youn HY (2018) Treatment of solid tumors in dogs using veterinary high-intensity focused ultrasound: A retrospective clinical study. Vet J 234, 126-129.
- Salyer SA, Wavreille VA, Fenger JM, Jennings RN, Selmic LE (2020). Evaluation of microwave ablation for local treatment of dogs with distal radial osteosarcoma: A pilot study. Vet Surg 49, 1396-1405.
- Seward MC, Daniel GB, Ruth JD, Dervisis N, Partanen A, Yarmolenko PS (2019) Feasibility of targeting canine soft tissue sarcoma with MR-guided high-intensity focused ultrasound. Int J Hyperthermia 35, 205-215.
- Shipov A, Kelmer G, Lavy E, Milgram J, Aroch I, Segev G (2015) Long-term outcome of transendoscopic oesophageal mass ablation in dogs with Spirocerca lupi-associated oesophageal sarcoma. Vet Rec 177, 365.
- Ter Haar G (2016) HIFU Tissue Ablation: Concept and Devices. Adv Exp Med Biol 880, 3-20.
- Vascellari M, Baioni E, Ru G, Carminato A, Mutinelli F (2009) Animal tumour registry of two provinces in northern Italy: incidence of spontaneous tumours in dogs and cats. BMC Vet Res 5, 39.
- Vlaisavljevich E, Greve J, Cheng X, Ives K, Shi J, Jin L, Arvidson A, Hall T, Welling TH, Owens G, Roberts W, Xu Z (2016a) Non-Invasive Ultrasound Liver Ablation Using Histotripsy: Chronic Study in an In Vivo Rodent Model. Ultrasound Med Biol 42, 1890-902.
- Vlaisavljevich E, Kim Y, Owens G, Roberts W, Cain C, Xu Z (2014) Effects of tissue mechanical properties on susceptibility to histotripsy-induced tissue damage. Phys Med Biol 59, 253-70.
- Vlaisavljevich E, Maxwell A, Mancia L, Johnsen E, Cain C, Xu Z (2016b) Visualizing the Histotripsy Process: Bubble Cloud-Cancer Cell Interactions in a Tissue-Mimicking Environment. Ultrasound Med Biol 42, 2466-77.
- Weisse C, Berent A, Solomon S. Combined transarterial embolization, systemic cyclophosphamide, and cryotherapy ablation for “Hi-Lo” maxillary fibrosarcoma in a dog. In: Proceedings of the 8th Annual Meeting, Veterinary Endoscopy Society 2011, San Pedro, Belize.
- Xu W, Zhang X, Hu X, Zhiyi C, Huang P (2019) Translational Prospects of ultrasound-mediated tumor immunotherapy: Preclinical advances and safety considerations. Cancer Lett 460, 86-95.
- Xu Z, Hall TL, Vlaisavljevich E, Lee FT, Jr. (2021) Histotripsy: the first noninvasive, non-ionizing, non-thermal ablation technique based on ultrasound. Int J Hyperthermia 38, 561-575.
- Yang T, Case JB, Boston S, Dark MJ, Toskich B (2017) Microwave ablation for treatment of hepatic neoplasia in five dogs. J Am Vet Med Assoc 250, 79-85.
- Yas E, Kelmer G, Shipov A, Ben-Oz J, Segev G (2013) Successful transendoscopic oesophageal mass ablation in two dogs with Spirocerca lupi associated oesophageal sarcoma. J Small Anim Pract 54, 495-8.
Corresponding author:
Kelsey Murphy
e-mail: This email address is being protected from spambots. You need JavaScript enabled to view it.